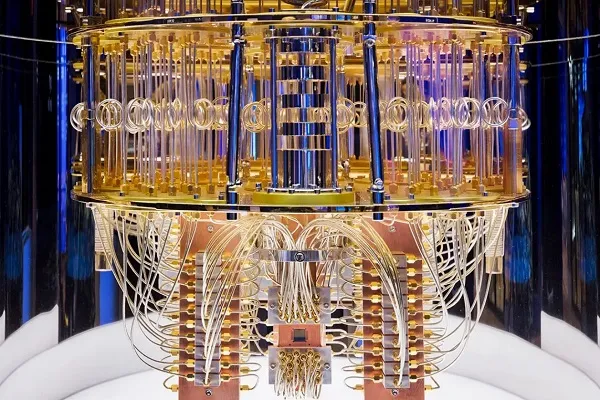
Classical Mechanics: Larger-than-atomic particles are the focus here. Newton's principles of motion and Maxwell's theory of electromagnetic waves serve as its foundation. Continuous emission or absorption of energy of any magnitude is possible. Positions and velocities give a precise definition of the system's state. It is possible to foretell the future condition with absolute certainty.
Quantum Computing: Particles on the nanoscale are the focus. This concept relies on the Schrodinger equation. According to Planck's hypothesis, the term "quantum" comes from the idea that only discrete amounts of energy may be emitted or absorbed. It is impossible to precisely specify a system's state due to Heisenberg's uncertainty principle and the dual nature of matter (both particle and wave) postulated by the de Broglie hypothesis. It provides the odds of discovering particles at various points in the universe. This theory is probabilistic, and it has been subjected to rigorous experimental testing. Measurement results for quantum systems are not guaranteed.
In the field of computation known as combinatorics, where the order of data is crucial to finding the best solution, digital computing has its constraints. Even the most powerful computers may struggle to keep up with the iterative complexity of these calculations. Software and hardware based on the principles of quantum mechanics may be able to execute combinatorics and other calculations at significantly higher speeds. Cybersecurity, Bioengineering, AI, Finance, and sophisticated manufacturing are just few of the potential fields of use. In the field of computation known as combinatorics, where the order of data is crucial to finding the best solution, digital computing has its constraints. Even the most powerful computers may struggle to keep up with the iterative complexity of these calculations. Software and hardware based on the principles of quantum mechanics may be able to execute combinatorics and other calculations at significantly higher speeds. Major Breakthrough in Developing Quantum Computers That Can Solve Critical Challenges of Our Time. For the first time, scientists from the University of Sussex and Universal Quantum have successfully pieced together quantum microchips like a Jigsaw Puzzle to create robust quantum computers, setting new benchmarks for connection speed and accuracy in the process.
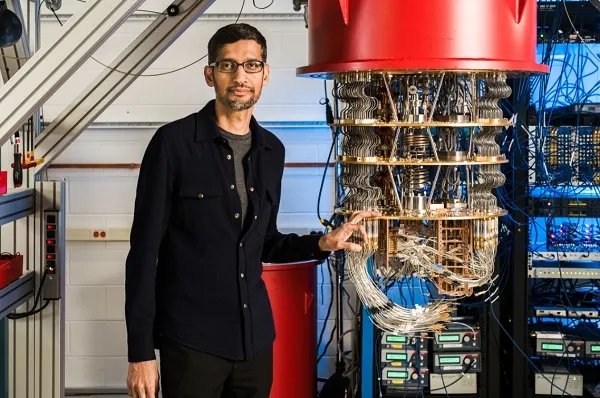
The largest quantum computer currently in use has 100 qubits. The most powerful supercomputers of today can't begin to tackle the complex challenges that experts believe would require millions of qubits to solve. There is a worldwide "quantum race" to develop quantum computers that can aid in a wide range of critical social issues, from medication discovery to more energy-efficient fertilizer manufacturing and the resolution of crucial issues in practically every industry, from aviation to finance. In a world-first demonstration of record-breaking speed and precision, researchers from the University of Sussex and Universal Quantum have shown that quantum bits (qubits) may be transferred directly across quantum computer microchips. Traditional computers use binary bits, which can only be either 0 or 1, to store and process data. Each of these two values may be represented by one of the billions of transistors found in a standard laptop. Classical computers come in a broad variety of sizes and processing capacities, but they are all based on the same fundamental principle of binary logic. Quantum computers are distinctive in many essential ways. Each of its quantum bits, or qubits, can be in one of three states: 0, 1, or a hybrid of the two. That's because of the superposition principle in quantum mechanics. In quantum mechanics, a particle can behave as if it were in two places at once.
The superposition state of a qubit is extremely delicate. Qubits are susceptible to error and the loss of quantum information due to minute environmental disturbances or material flaws. The useful lifetime of a qubit is capped by this phenomenon, known as decoherence. Using a precisely timed pulse of electromagnetic radiation to exert control over the qubit and carry out logical operations. Decoherence can be caused by unintentional electromagnetic noise produced during this manipulation procedure. A trapped ion quantum computer, which exploits individual atoms and their intrinsic quantum mechanical behavior as qubits, is one solution to the problem of manipulating qubits while keeping them protected from decoherence. As opposed to their supercooled counterparts, qubits made from atoms are much easier to implement. The concept of neutrons, electrons, and protons, and their related properties, was gradually becoming central to atomic model comprehension. Including ideas like matter being made up of atoms and atoms existing in specific integer ratios, John Dalton developed his atomic theory. Quantum computing requires an alternative theoretical framework for its operations. A state of an item is precisely specified in classical physics. Until we witness an object, it does not exist in a definite quantum mechanical state. The probabilities of the states of two things and their connections are open to debate. Non-binary qubits of information (as opposed to binary bits) are used to record and store data in quantum computers, reflecting the quantum world's many possible states.
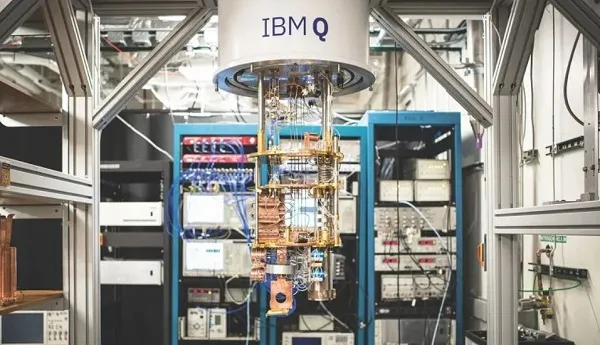
It is extremely challenging to construct quantum computers. The physicists, technologists, and materials scientists who are trying to carry out quantum operations on the many candidate qubit systems that exist on the size of single atoms must continually balance two competing needs. The environment must first be kept away from qubits to preserve their delicate quantum states, which are required for computation. When a qubit maintains its desired state for a longer period of time, this is referred to as its "coherence time" The value of solitude is emphasized in this view. Second, qubits need to be entangled, shuffled around physical architectures, and programmable in order to execute algorithms. The more accurately one can perform these procedures, the higher their "fidelity" It's challenging to find a happy medium between the time spent alone and with others. With the advent of quantum computing, hitherto intractable issues in classical computation may finally find an answer. Progress is being made, but yet no quantum computer is advanced enough to perform calculations that a classical computer cannot. In recent years, billions of dollars have been invested in quantum computing due to the possibility that it may speed up and reduce the cost of solving large-scale combinatorics issues. The most promising avenue may lie in developing even more novel uses for the quantum solutions now available. Due to its tremendous vulnerability, a quantum system requires complete isolation. The decoherence time that is longer than the algorithm's execution time is difficult to achieve.